Tunneling dynamics of a surface hydroxyl group
08/02/09 09:17 Filed in: single molecules, STM
Briefing of a recent publication: "Tunneling dynamics of a hydroxyl group adsorbed on Cu(110)", PRB 79, 035423 (2009).
Structure and dynamics of a hydroxyl (OH) group on the Cu(110) surface is reported in this paper. The inclined OH axis of the hydroxyl group switches back and forth between the two equivalent orientations. Additionally, hydroxyl group is a basic system containing hydrogen. Hydrogen is the simplest element and shows quantum effects due to its low mass. Quantum tunneling of hydrogen atoms (or protons) plays crucial roles in diverse chemical, physical, and biological process. In this work, we investigated the dynamics of hydroxyl spices, including tunneling effect of hydrogen bound by double minimum potential well, as shown in the right figure.
[Fig. 1] An isolated hydroxyl group on the surface can be artificially-produced by using an STM. (a)-(b) STM images of the generation of OH. A voltage pulse of 2 V for 0.5 s was applied to a water monomer imaged as a round protrusion in (a). The product (OH) appears as paired depressions. The reaction competes with translation of the parent water monomer, and the product is usually observed away from the original position. (c) The relative position of OH to nearby water monomers located on a top of Cu atoms. The grid lines represent the lattice of the Cu(110) surface.
[Fig. 3] A remarkable isotope effect was observed for dynamical properties of the hydroxyl species. (a) STM images for OH and OD on Cu(110). There are not much difference in the image between OH and OD. (b) Only in the case of OD, the two-state fluctuation in the tunneling current is observed with the tip fixed over the one depression. The fluctuation is not observed for OH. (c) Schematic of the hydroxyl switching between the two orientations under the tip. We tentatively assigned the high-current (low-current) states to the orientations of OD pointing toward (away from) the tip.
[Fig. 4] (a) The calculated structure for the hydroxyl species. It shows that the molecular axis is inclined along [001] direction. (b) Calculated potential-energy surface of OH as a function of the lateral displacements. The two minima correspond to the inclined geometries, and the potential barrier between the minimum and the saddle point is 0.14 eV, which is too high to be overcome by thermal process at 6 K.
[Fig. 5] (a), (b) I-V and d2I/dV2 spectra (IETS) measured over the one depression of hydroxyl species. The peak (dip) is observed for OH and OD at positive (negative) bias voltages. The inset shows fractional occupation of the high-current state for OD plotted as a function of bias voltage. The switch motion is enhanced and the fractional occupation increases abruptly at the onset voltage that coincides with the peak in IETS. This suggests that the vibration excitation couples with the switch motion, which causes the change of the occupation of two-state system.
[Fig. 6] (a) The spatial distribution of the IETS peak intensity for OH. At each pixel, the feedback was disabled at the tip height to give 5 nA at 24 mV. (b) The simultaneously acquired topographic image at 24 mV and 5 nA. The image size is 11 × 14 Å2. What’s interesting is that both the peak and dip appear depending on the lateral position of the tip over the same molecule.
2009/02/06 T. Kumagai
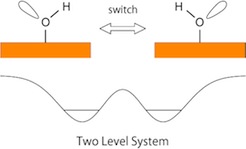
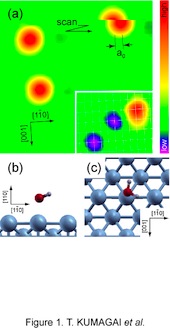
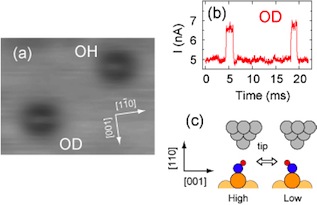
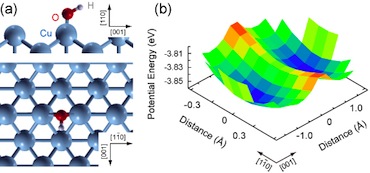
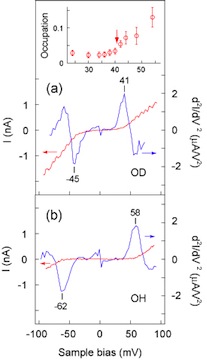
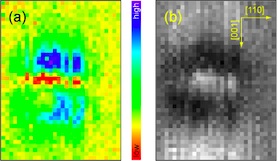
2009/02/06 T. Kumagai